Before I get into the nitty grities of the physics of the Faraday cage and the ice bucket experiment, I would like to start with a simple demonstration of the cage that I did with things around the house that I think is pretty amazing.
Table Of Contents
Simple At-Home Faraday Cage Experiment
History of Induction and Conduction
Benjamin Franklin to Coulomb to Faraday
The Gold-Leaf Electrometer
Faraday’s 1843 Ice Pail Experiment EXPLAINED
Faraday Cage vs. Static Electric Fields
From Faraday to Maxwell to Heaviside to Hertz
How a Faraday Cage Protects against Radio
Faraday Cages for Microwaves and High Frequency
References
Video Script
Simple At-Home Faraday Cage Experiment
So, take an AM/FM radio and put it into a metal container. Then add tin foil to the top and voila – the radio music is cancelled. Amazingly this works even if the container has holes, and the holes can be really big.
How does it work? Radio can make it through solid walls but not past tin foil with holes in it? Why does it protect you from lightning, block some electromagnetic waves like radio or microwave but not the visible light (which is also an electromagnetic wave)? Ready to learn in detail how the cage works starting and also about Faraday’s famous ice pail experiment of 1843 and how it relates to his cage experiment of 1837 as well as an in-depth study of induction?
History of Induction and Conduction
When Faraday created his cage in 1837, he created a new concept that he called “lines of inductive force” to describe how it worked. Now when most people hear “induction” or “inductive force” we think of what is called “magneto-electric induction,” discovered by Michael Faraday where changing the magnetic field in a coil induces current in the coil.
However, that is not what Faraday was referring to here. Instead, he was talking about static electric induction (or moving charges without touching) which predated that discovery by over 100 years! In fact, induction and conduction were both discovered in the same year 1727, by the same guy, a retired clothing dyer named Stephan Gray.
See, Gray was an amateur scientist with some powerful friends [John Flamseed: the royal astronomer] and some even more powerful enemies, including Sir Isaac Newton and Newton’s assistant Francis Hauksbee who kept Gray from publishing and working on physics full time.
When Hauksbee died in 1713, Newton’s new assistant John Desaguliers quietly helped Gray behind the back of his ailing boss, which is how Gray ended up with a pension at an old age home for deserving soldiers, even though he had never been a soldier! When Newton died in 1727, Gray was free to publish without resistance, he just needed to discover something new, which he did over 2 years later on July 8, 1729.
That was the day that Gray discovered that if he rubbed a glass tube with a stoper in it, feathers would be not only attracted to the charged glass, they would also be attracted to the stoper.
In other words, Gray discovered that electricity can move or conduct. When Gray attempted to make the electricity move longer and longer distances, he found that if he tied up his line with silk, he could get the “electric virtue” to flow long distance but metal wires would let the “electric virtue” flow away.
Thus, he discovered that all materials that he found could be placed into two categories: materials that would allow the “electric virtue” to flow (conductors) and materials that would prevent the “electric virtue” from flowing (insulators). Note that the terms “insulator” and “conductor” were actually created a few years after Gray’s experiments by his friend John Desaguliers, although their meanings took a few years to settle into our current definitions.
Then, less than a month later, on August 5, 1729, Gray was conducting an typical experiment where he electrified a rod and then placed it on a line to see feathers and fluff attract at the end of the line.
This time, however, Gray discovered that the feathers were attracted to the line before the charged rod touched it. In other words, the electricity was induced to flow in the line without touching. For this reason this was called induction, although once again, the name took a while to settle down to the current definition.
Benjamin Franklin to Coulomb to Faraday
Amusingly, Gray then came up with the wild demonstration of tying up a young boy with silk and placing a charged rod near his feet and watching as pieces of fluff went to his body.
This odd electric party trick kicked off decades of wild elaborate “electricity parties” which led to a plethora of new electrical discoveries. If that picture looks familiar, it is because I love it so much that I used it in the cover of my new book.
Anyway, one of the people who was inspired to join the “electric party” was a 40-year-old Benjamin Franklin in 1746.
In his electrical parties Franklin decided that electricity wasn’t created or destroyed only moved and named the two types of electricity positive and negative so that if you have equal amounts of both then the total charge adds to zero.
Franklin also flew a kite in a thunderstorm and, more importantly for the discovery of the Faraday cage, accidentally discovered in 1755 that the interior of a charged metal jar had no charges or electric forces on the inside, a result that confused Franklin.
26 years later, in 1786, Charles Augustin Coulomb, of Coulomb’s law fame examined charges with his sensitive electroscope and found the same, that a charged object has its charges on the surface and the interior was protected from electric forces.
50 years later in 1836, Faraday read Coulomb’s paper and was inspired to try it out himself.
That is why, in 1837, Faraday upscaled Coulombs experiment and built a giant cube covered in every direction in copper wire and studied the electrical effects inside the cube while experiments were conducted outside the cube.
The Gold-Leaf Electrometer
Note that at the time, the most precise electric equipment available was called a gold-leaf electrometer, which is two thin pieces of gold in a glass case (to block air currents) and connected with metal to a plate on the top.
The idea is that if you placed a charged object on or near the plate, the gold would become charged with the same charge and then repel each other. The more charge, the more they repel.
This is why, Faraday recalled that: “I put a delicate gold-leaf electrometer within the cube, and then charged the whole by an outside communication, very strongly, for some time together; but neither during the charge [nor] after the discharge did the electrometer or air within show the least signs of electricity.”
This cube is now called a Faraday cage, but how did it work? Faraday said it worked by electrical induction (moving charges without touching as discovered by Gray).
Specifically, Faraday declared that his cage worked because all electrified objects create something that he called “line[s] of inductive force,” and that metals and conductors let electricity flow easily to cancel out these lines, and insulators do not.
We still think this today but we now call “lines of inductive force” Electric fields! (Yes, Faraday did create the idea of both magnetic and electric fields, amazing right?).
However, the collective scientific world was less than enthusiastic about this way of thinking and soon Faraday was in debates with multiple scientists who politely tried to get Faraday to see the errors of his ways.
Faraday had trouble standing up for his point of view as by the late 1830’s Faraday’s health started to falter. Doctors didn’t know what was wrong with him, but it seems likely he was suffering from memory loss and confusion due to handling heavy metals in the 1820s without protective materials.
In fact, his health kept him completely away from science between early 1841 and 1843. It was only in late January, 1843 that he could even start to read science literature again, even for a bit.
Faraday’s 1843 Ice Pail Experiment
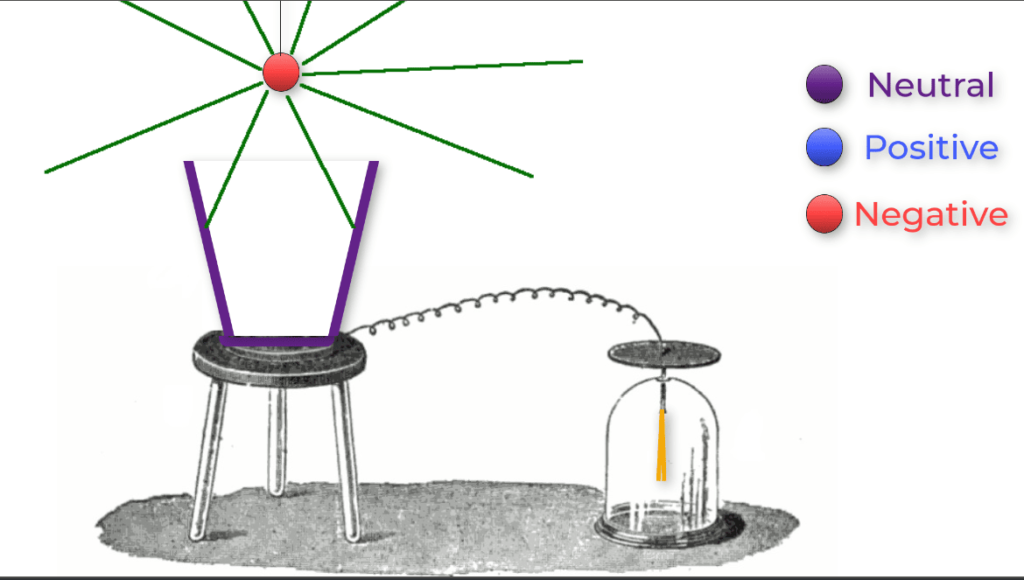
Figure a: “Still above the bucket” Faraday started with a charged ball (in red) above a neutral ice bucket (purple). The Electric field lines (in green) don’t affect the bucket much
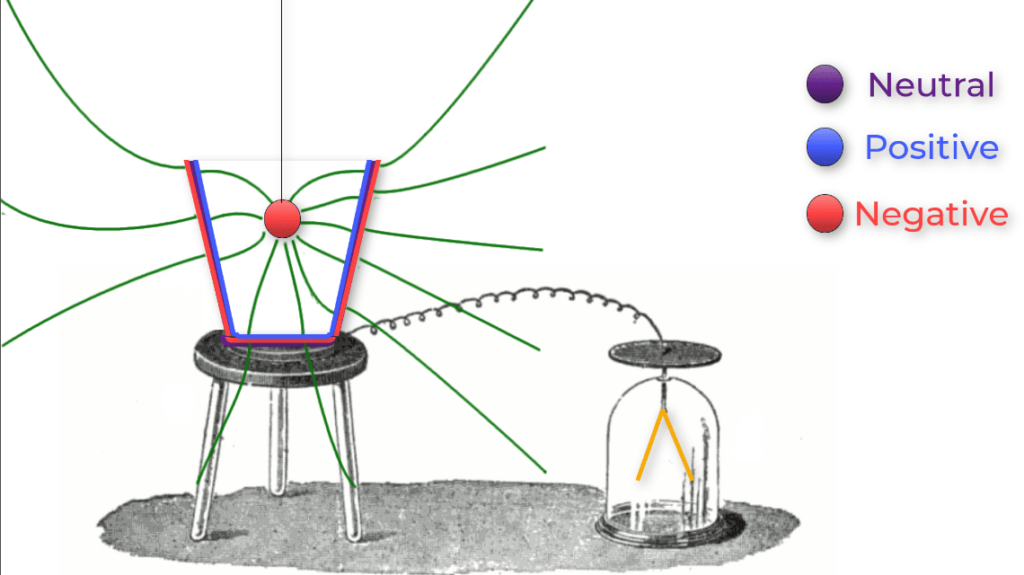
Figure b: “just in bucket E field” When the charged ball (red) enters the neutral ice bucket the electric field (in green) pushes the electrons in the bucket so that the outer surface has the same charge as the ball and the inner surface has the opposite charge as the ball (blue) so that the electric field in the conductive bucket is zero
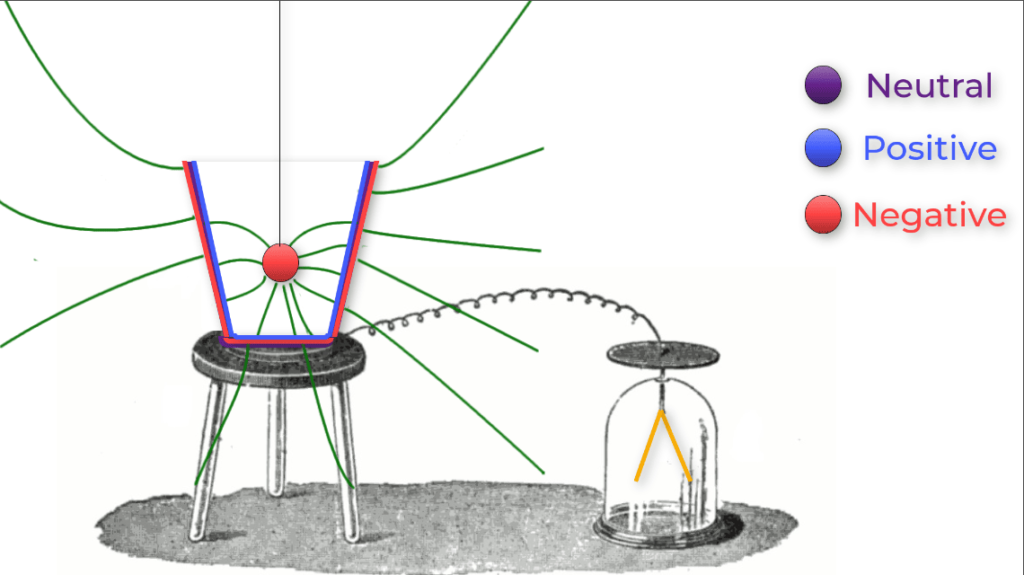
Figure c: “More in ice bucket” & “far in bucket” As the charged ball (red) moves inside the bucket, the charges on the inner surface coalesce near the ball while the charges on the outer surface remain evenly distributed.
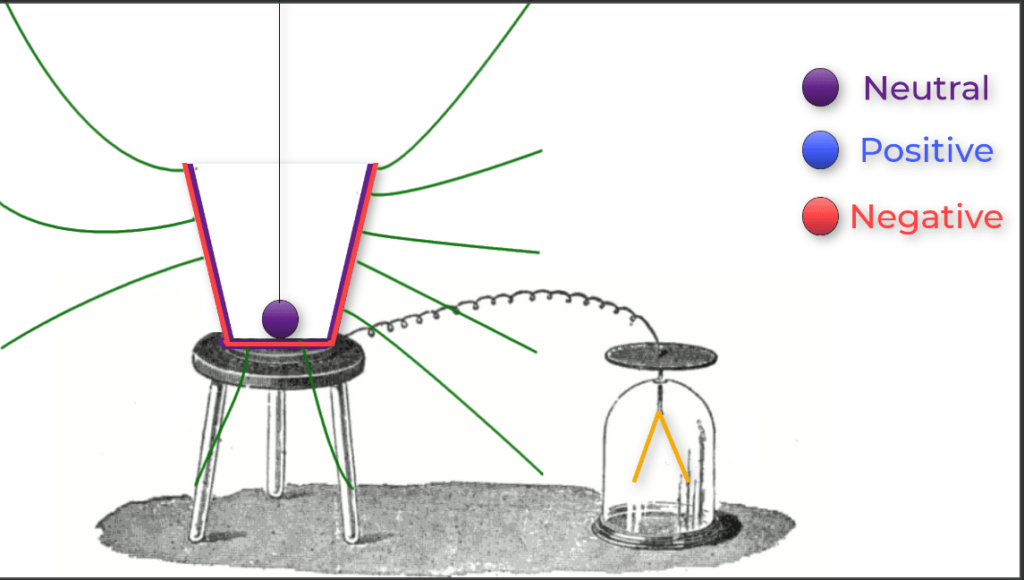
Figure d: “Touching bucket”: If the charged ball touches the inner surface electrons move so that the opposite charges cancel out leaving the ball and the inner surface of the bucket neutral (purple). In this way, the inner surface of the bucket are shielded from the electric field from the outside charges.
It was at this time, in February of 1843, that Faraday conducted an experiment with an ice bucket that he said, “are the expression and proof of certain parts of my view of induction.” See, Faraday knew from the experiment by Benjamin Franklin in 1755 that if you charged up a conductive cup then the inside would be shielded from electric forces and even if you touched the inner surface of the container, you still would not gain a charge, a result that confounded Franklin.
To Faraday’s mind, this all made sense by thinking about rays of inductive lines of force (electric fields), but this train of thought wasn’t very clear to anyone else.
For that reason, Faraday took Franklin’s 1755 experiment and turned it on its head. He took a metal container (which was an ice bucket, thus the name), put it on an insulating stand and charged the ball instead of the bucket.
He then connected the outside of the bucket to a “gold leaf electrometer.” With the electrometer, Faraday could tell that when the charged ball went into the bucket, the outside of the bucket became electrified like the ball was.
If the ball was then removed, the bucket lost all electrification. This proved to Faraday that the charges in the bucket were electrically induced (or moved without touching).
Faraday also found that, if the charged ball touched the inside of the bucket, the outside charge stayed the same but the ball lost all of its charge.
Let’s look at this from a microscopic point of view. Imagine the ball had a negative charge, that would mean that it had too many electrons.
When the charged ball is lowered into the bucket, the electric field lines cause some electrons in the bucket move away from the ball until the extra charge on the outer surface is the same as the charge on the ball.
These leaves the inner surface of the bucket with a net positive charge equal to the charge on the ball (remember, charge is conserved). As the ball is moved towards the inner surface the electrons in the inner surface move so that most of it was neutral but the part closest to the ball is where most of the positive charge is nearest the ball.
For the ball, the extra electrons are attracted to the positive inner surface and collect closer to that point, leaving the rest of the ball neutral.
When the ball touches the inside of the bucket, all the extra electrons in the ball flow into the positive inner bucket, leaving it neutral and the outside of the bucket with all of the extra charge.
From this point on, the inside of the bucket is neutral and will not create any force or charge on a neutral object.
If a positive ball is used, the same process happens in reverse, once again leaving the ball neutral and the ice bucket with the net charge on the surface.
Either way, you are back to Franklin’s 1755 experiment, where a charge is on the outside of a charged conductor and a neutral ball is shielded from all electrical effects on the inside and the electric field on the inside of the conductor is always zero.
This is how a Faraday cage protects you from lightning, either natural or Tesla sparks.
In this case a burst of electrons flow between the cloud or the generator and the Faraday cage. The electricity then flows on the outside of the cage and then either into the ground or stays safely on the outside of the cage (if the cage is insulated), leaving the inside of the cage completely undisturbed.
This is true even if you are touching the inside of the cage, as long as your fingers don’t poke through the holes. In fact, one of the major reasons for the small holes for a Faraday cage in Tesla coil demonstrations is to keep your fingers inside, not to keep the lightning out. This is why you are safe in a metal airplane or a metal car if it is struck by lightning even though they have large insulating windows.
Faraday Cage vs. Static Electric Fields
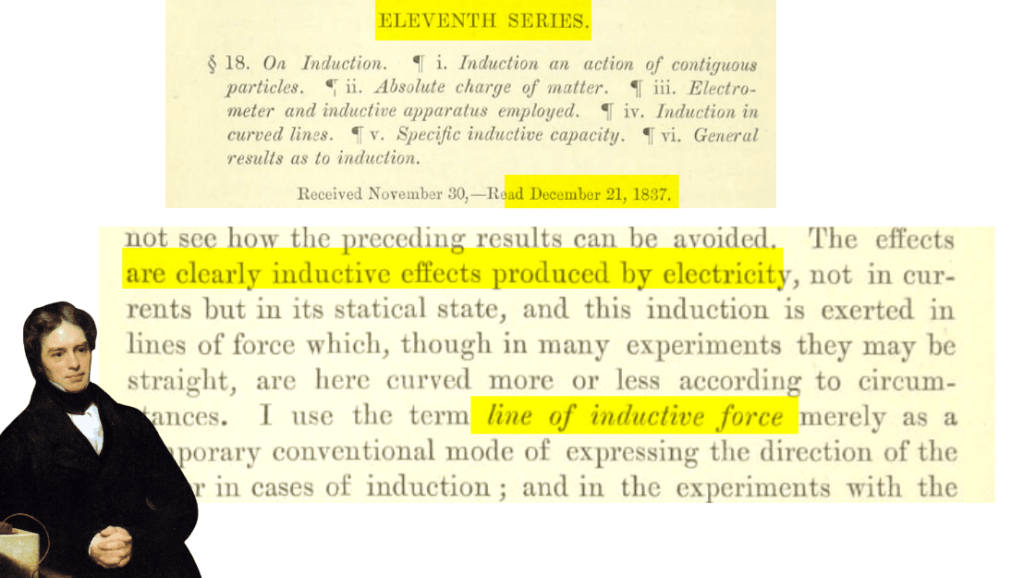
OK, but what happens if a net charge is *outside* of the Faraday cage, how does it shield you from the Electric Field? So, imagine that the Faraday cage is near a Van de Graff generator.
A Van de Graff generator has a motor that spins a rubber belt against a metal comb which collects extra electrons and leaves the metal sphere on the top with a positive charge and between 50 and 150 kVolts. If you stand next to a generator, some of the negative electrons in you will move towards the generator and leave the ends of your hair negatively charged.
If you have longish hair, the negative ends will then stick out from each other and towards the generator. Now imagine there is a Faraday cage, in that case, some electrons in the cage near the generator will move towards the positive generator and leave the other side positively charged.
In this case, the Faraday cage is neutral but it has a net positive charge on one side and a net negative on the other. This is arranged so that the net force on the inside of the cage is zero and, if you are in the cage, the electrons in your hair are not attracted to the generator as they are repelled by the negative charge on that wall as much as they are attracted to the generator.
In other words, some of the electrons in the conductor move so that the electric field in the conductor is zero. If the field isn’t zero, electrons will move until it is.
From Faraday to Maxwell to Heaviside to Hertz
But that still doesn’t explain how the cage protects you from waves of electric fields, specifically the electromagnetic waves of radio waves.
That has to do with Faraday too. See, as early as 1832, Faraday had the idea that magnetic forces and electric forces take time to travel in space and can make waves in the air, like, “the vibrations upon the surface of water, or those of air in the phenomena of sound…and most probably to light.”
By 1846, Faraday had the even more radical idea that not only could electric forces and magnetic forces make waves in air, maybe light itself is composed of vibrations of these forces (although Faraday thought this was a back and forth wave, or a lateral wave, not a up and down wave, called a transverse wave).
This idea was even more offensive to scientists than Faraday’s curved lines of force, and was almost universally ignored at the time. Fast forward 18 years later to 1864, when a 33-year-old mathematical physicist named James Clerk Maxwell wrote his masterpiece, “A Dynamical Theory of the Electromagnetic Field.”
In this paper, Maxwell took Faraday’s lines of force and connected them to all of the equations that were already there about the different electrical and magnetic effects.
In addition, Maxwell demonstrated that a transverse (or up and down) vibrating electric field would cause a transverse vibrating magnetic field that was 90 degrees from the electric field which travels at the speed of light, and that “light is an electromagnetic disturbance propagated through the field according to electromagnetic laws” 499
Not surprisingly, Maxwell’s equations were almost as controversial as Faraday’s idea’s 9 years earlier, but as the years passed, there grew a small but dedicated group of scientists and tinkerers who called themselves, “Maxwellians” and tried their best to tackle the complex mathematics of Maxwell’s ideas.
One Maxwellian was a young German scientist named Heinrich Hertz. One day in 1886, Hertz was showing his fiancée around his laboratory when he told her that one of his devices, called an induction coil, would make bursts of vibrating electric current, around 50 billion times a second.
Hertz’s fiancée Elizabeth wondered if it would make light as he had told her that vibrating electric current would make an electromagnetic wave.
Hertz told her it was far slower than light (which vibrates between 430 trillion and 750 trillion times per second), so although it should make a vibrating electric and magnetic field, it should make an *invisible* light wave.
That was why in 1887, Hertz added an antenna to the induction coil and discovered that it could be “caught” in a receiver and the wave did move at the speed of light.
Hertz had discovered radio waves (which is why frequency is now measured in Hz). In July of 1889, Hertz tested out his new wave on a faraday cage and found that, “not the slightest electrical disturbance would be detected in the wire in whatever direction waves were sent through the apparatus.”
How a Faraday Cage Protects against Radio
But how does it work? In a radio, an electric signal vibrating between 30 and 300 billion times a second is placed on an antenna. All electrons due to having a charge, create an electric field around them but by vibrating, these electrons create a vibrating electric field that emanates from them at the speed of light.
As moving electrons or an electrical current create a magnetic force, these vibrating electrons also make a vibrating magnetic field, which is why this is called an electromagnetic wave.
When the electric field from a radio wave hits the electrons in the Faraday cage, they react the same way as they did to the static electric field from a charged van de Graaff generator.
In other words, they move to cancel out the electric field in the cage. You could also think of it as the electrons on the surface of the conductor making their own EM waves that cancel out the EM wave in the cage.
Note that the cage isn’t destroying the wave, merely diverting it. Just in the example of the single static charge outside a cage, the electrons in the cage move so as to negate the electric field in the cage, but it still exists outside the cage.
For all or most of the radio waves to be cancelled, the cage needs to be completely surrounding the object so that all of the waves can work together.
However, the cage doesn’t need to be completely solid, it can have holes as long as the holes in the cage are significantly smaller than the wavelength of the wave (a rule of thumb that is often followed is to limit the holes to be smaller than 1/10th of the wavelength).
This is easy to do for radio waves as they have a wavelength of between 30 cm and thousands of meters. For example, the radio station 104.5 has a frequency of 104.5 Million Hz, and a wavelength of 2.8 meters, which means a cage with 28 cm (11 inch) holes should kill the signal.
This is even easier for most Tesla coils as they tend to operate on very low frequencies. For example, the “Megazapper” that I was zapped with while I stood in a Faraday cage ran at 112 KHz, which had an astonishing wavelength of 2,678 meters (or 1.66 miles)!
However, as I said, the big danger of Tesla coils is being hit with that lightning which can be deadly, so the Faraday cage is designed to have holes that are too small for even a pinky finger to get through.
Faraday Cages for Microwaves and High Frequency
As the frequency increases, the wavelength and the size of the holes allowed decreases.
For microwaves that heat your food tend to be at 2.45 gigahertz and therefore have a wavelength of about 12 cm (4.7 inches) which is why the grating on your microwave ovens can have little holes in them and still protect you from getting cooked by their EM waves.
By the time you get to infrared, the holes would be so small that most people don’t bother as you can’t see through them so the Faraday cage to protect against these tend to be solid. Even then, they have to be careful as there are often leaks that you don’t see.
Just like if you think a room is lightproof, once your eyes get adjusted to the dark you can see that light makes it past cracks that you didn’t see before.
Note that Faraday cages are due to the movement of electrons, so they are an electric effect.
Because of this they can protect against static electric fields and varying electric fields as electric fields create a force on electrons.
They can also protect against varying magnetic fields as a changing magnetic field can move an electron and make a current, just as Faraday discovered in 1831.
However, Faraday cages cannot shield against static magnetic fields, which is why a compass works fine inside them an airplane.
So that is the Physics of the Faraday cage. I have made a ton of videos, including on a more detailed history of the cage and its influence as well as ones on Faraday, Maxwell and Hertz. I especially think that my video on Maxwell needs some love.
However, I realized that I haven’t yet done a video on Oliver Heaviside and how he made the modern version of Maxwell’s equations, and that is next time on the Lightning Tamers.
If you liked learning the history with the real science you will love my new book “The Lightning Tamers” which is available on October 12, 2022 in ebook, paperback, and hardback and available today to preorder link below.
References
Audrey T Carpenter John Theophilus Desaguliers (Bloomsbury Publishing, 2011), 64.
Stephen Gray, “A Letter to Cromwell Mortimer,” Philosophical Transactions 37, no. 417 (1731): 20, https://doi.org/10.1098/rstl.1731.0005.
John L. Heilbron, Electricity in the 17th and 18th Centuries: A Study of Early Modern Physics (Berkeley, CA: University of California Press, 1979), 292.
Stephen Gray, “A Letter to Cromwell Mortimer,” Philosophical Transactions 37, no. 417 (1731): 34
Ibid, 39.
Benjamin Franklin, Experiments and Observations on Electricity (London: E. Cave, 1751), 2.
Ibid. 6.
Ibid. 326
Ibid. 366.
Michael Faraday, “Experimental Researches in Electricity – Series 11” (Dec 21, 1837) Proceedings of the Royal Society vol 128 (1838), 20
Faraday to Dr. Hare (March 11, 1843) Experimental Researches in Electricity – vol 2 (1844), 274. “prevented me from working or reading in science for the last two years”
Faraday “On Static Electrical Inductive Action” (Feb, 1843) Experimental Researches in Electricity – vol 2 (1844), 279.
Michael Faraday “On Static Electrical Inductive Action” (Feb 4, 1843) Philosophical Magazine vol. 22, 200.
Sealed Note Faraday to John Children (March 12, 1832) The Correspondence of Michael Faraday vol. 2 (1991), 26
Heinrich Hertz “On the Propagation of Electric Waves through Wires” (Originally published in German in July, 1889 translated Aug, 1889) Philosophical Magazine vol. 28 (July-Dec 1889), 122. Also check out Oliver Lodge “On Lightning-Guards for Telegraphic Purposes” (April 24, 1890), Proceedings of the Institution of Electrical Engineers vol. 19 (1891), 376.